Quantum simulator realizes strongly interacting Mott-Meissner phases in bosonic flux ladders
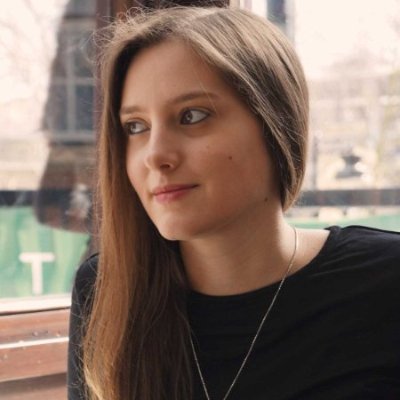
Ingrid Fadelli
contributing writer
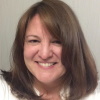
Lisa Lock
scientific editor
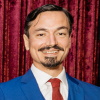
Robert Egan
associate editor

When exposed to periodic driving, which is the time-dependent manipulation of a system's parameters, quantum systems can exhibit interesting new phases of matter that are not present in time-independent (i.e., static) conditions. Among other things, periodic driving can be useful for the engineering of synthetic gauge fields, artificial constructs that mimic the behavior of electromagnetic fields and can be leveraged to study topological many-body physics using neutral atom quantum simulators.
Researchers at Ludwig-Maximilians-Universität, Max Planck Institute for Quantum Optics and Munich Center for Quantum Science and Technology (MCQST) recently realized a strongly interacting phase of matter in large-scale bosonic flux ladders, known as the Mott-Meissner phase, using a neutral atom quantum simulator. Their paper, in Nature ÃÈÃÃÉçÇøics, could open new exciting possibilities for the in-depth study of topological quantum matter.
"Our work was inspired by a long-standing effort across the field of neutral atom quantum simulation to study strongly interacting phases of matter in the presence of magnetic fields," Alexander Impertro, first author of the paper, told ÃÈÃÃÉçÇø. "The interplay of these two ingredients can create a variety of quantum many-body phases with exotic properties.
"While their microscopic mechanisms are typically well understood, the emergent many-body properties are elusive and hard to probe in conventional solids, with a notable example being the (fractional) quantum Hall effect. Unfortunately, it turned out that the Floquet engineering technique, which is one of the primary methods for obtaining an effective magnetic field for neutral atoms, generally causes strong heating in interacting quantum systems."
The heating processes prompted by Floquet engineering techniques are known to rapidly destroy fragile quantum states. As a result, most previous experiments probing exotic quantum many-body phases only focused on non-interacting or weakly interacting systems, while strongly-correlated ones remained limited to only two particles.
The first objective of the recent study by Impertro and his colleagues was to leverage the capabilities of a new experimental quantum simulation platform they developed to realize quantum many-body states with artificial magnetic fields and strong interactions producing little heating. In addition, they hoped to simulate larger systems that reached well beyond the two-particle systems realized in previous experiments.
In their experiments, the researchers utilized optical superlattices, a short-spacing vertical lattice and a so-called Feshbach resonance that provides an important tuning knob. In addition, they employed a recently developed technique for the precise measurement of particle currents.
"Using the optical superlattices, we partitioned a two-dimensional optical lattice into an independent array of ladder systems, in which we realize the experimental studies," explained Impertro. "Additionally, the double-wells that form the rungs of the ladders are also the basis for the Floquet engineering technique that we use to create an artificial magnetic field.
"Intuitively, the technique modifies the motion of particles in the lattice using additional laser beams, which in turn imprint the effect of a magnetic field onto the atoms to mimic a Lorentz force or Hall deflection."

Finally, Impertro and his colleagues leveraged the Feshbach resonance in cesium. This property of cesium allowed them to tune the interaction strength between atoms over a wide range, which is important both for preparing the desired strongly interacting quantum states with low heating and to probe the response of the quantum states to a changing interaction strength.
"The central challenges we encountered when preparing the states were to find suitable parameter regimes where the heating rate due to the periodic modulation (Floquet engineering) is minimal, which is particularly challenging for large many-body systems, and to find preparation paths that allow us to adiabatically transform an easy-to-prepare initial state into the quantum state of interest, without creating excitations," said Impertro.
"Lastly, a central quantity that characterizes the ground states of flux ladders, such as the Mott-Meissner phase, are persistent particle currents."
Notably, quantum gas microscopes like the one employed by Impertro and his colleagues can typically only measure local densities and fail to measure currents. To enable the measurement of currents, the team employed a current detection technique that they developed as part of their earlier studies, adapting it for the purpose of their experiment.
"For the first time, we were able to prepare low-temperature states in Floquet engineered quantum systems with a large number of particles and microscopically study their properties," said Impertro. "We also demonstrated the measurement of particle currents with full spatial resolution across large systems, which constitutes an entirely new way to probe these physics using quantum gas microscopy.
"This constitutes a key step towards studying fractional quantum Hall phases in synthetic quantum systems, which is a longstanding goal in various communities, ranging from superconducting qubits to photonics, neutral atoms and Rydberg atom arrays."
Impertro and his colleagues hope that their recent efforts will inform future theoretical and experimental studies focusing on topological many-body physics. In the future, the methods they devised could help to realize other complex quantum phases that have so far proved difficult to engineer experimentally.
"On the one hand, we show that it is now indeed feasible to experimentally realize interacting systems with an artificial magnetic field and reach significant system sizes," said Impertro. "This offers a new playground for quantum simulation of many-body systems in- and out-of-equilibrium in regimes that are extremely difficult to access with classical numerical techniques. On the other hand, a comparison with numerical simulations allowed us to extract an estimate of the effective temperature of the prepared states."
The new methods introduced by Impertro and his colleagues could soon enable the validation of theoretical models of strongly interacting quantum many-body systems, while also potentially contributing to the future advancement of quantum technologies. In their next studies, the researchers plan to further explore the rich phase diagram of interacting flux ladders beyond the Mott-Meissner phase, for instance by probing vortex or symmetry-broken states.
"In these future studies, it will be central to reduce the experimentally accessible temperature scales further, as many of these phases are even more fragile," added Impertro. "Additionally, a long-term goal is to extend the ladder geometry to full-2D systems, where exotic physics such as anyons in fractional quantum Hall states can be studied."
More information: Alexander Impertro et al, Strongly interacting Meissner phases in large bosonic flux ladders, Nature ÃÈÃÃÉçÇøics (2025). . On arXiv:
Journal information: Nature ÃÈÃÃÉçÇøics , arXiv
© 2025 Science X Network